Feeding habits of Agromyzidae
All Agromyzidae larva are feeders on plant tissue and are mostly known as leaf miners but they also utilise all other parts; stems, seeds, roots and in the case of trees, young trunks and twigs, whilst species in the genus Hexomyza form twig-galls. The majority of species are monophagous, being dependant on one single plant or several species within the same genus. On the other hand, some are oligophagous, feeding on a number of genera within a single family or on several related families of the same order. For example, Melanagromyza aenea is strictly monophagous, being restricted to feeding only on Urtica, whereas Agromyza albipennis feeds on several genera of Gramineae, therefore being oligophagous. Very few species are polyphagous, feeding on a wide range of unrelated host plants. One example of a highly polyphagous species is Liriomyza strigata, which is known from over 240 host plant genera, in over 35 families, worldwide.
The video below shows a Phytomyza obscurella larva feeding, using its mandible to ‘scrape’ away the plant tissue;
Phytomyza obscurella Fallén, 1823 larva feeding action © Barry P. Warrington
Agromyzid larvae by Graham E. Rotheray
The ease of finding stem and leaf mines makes the Agromyzidae one of only a handful of cyclorrhaphan families whose early stages are reared on a routine basis. Unfortunately, rearing tends to by-pass larvae as subjects of study and what we know about them is rudimentary although indicative of a rich source of information (Dempewolf 2001). The following characters appear to distinguish mining if not other types of agromyzid larvae (Ferrar 1987, Smith 1989):
- mandible vertically-orientated with forward facing hooks (Fig. 1);
- prothorax with anterior spiracles close together at the dorsal midline (Figs. 2& 3).
Widespread agromyzid larval characters not confined to them include:
- head skeleton with intermediate and basal sclerites smoothly adjoined (arrow, Fig. 1);
- dorsal cornu longer than ventral cornu (Fig. 1);
- body segments with rings of red to black micro-hooks or spicules (Fig. 2);
- anus bordered by a pair of fleshy projections (Fig. 5).
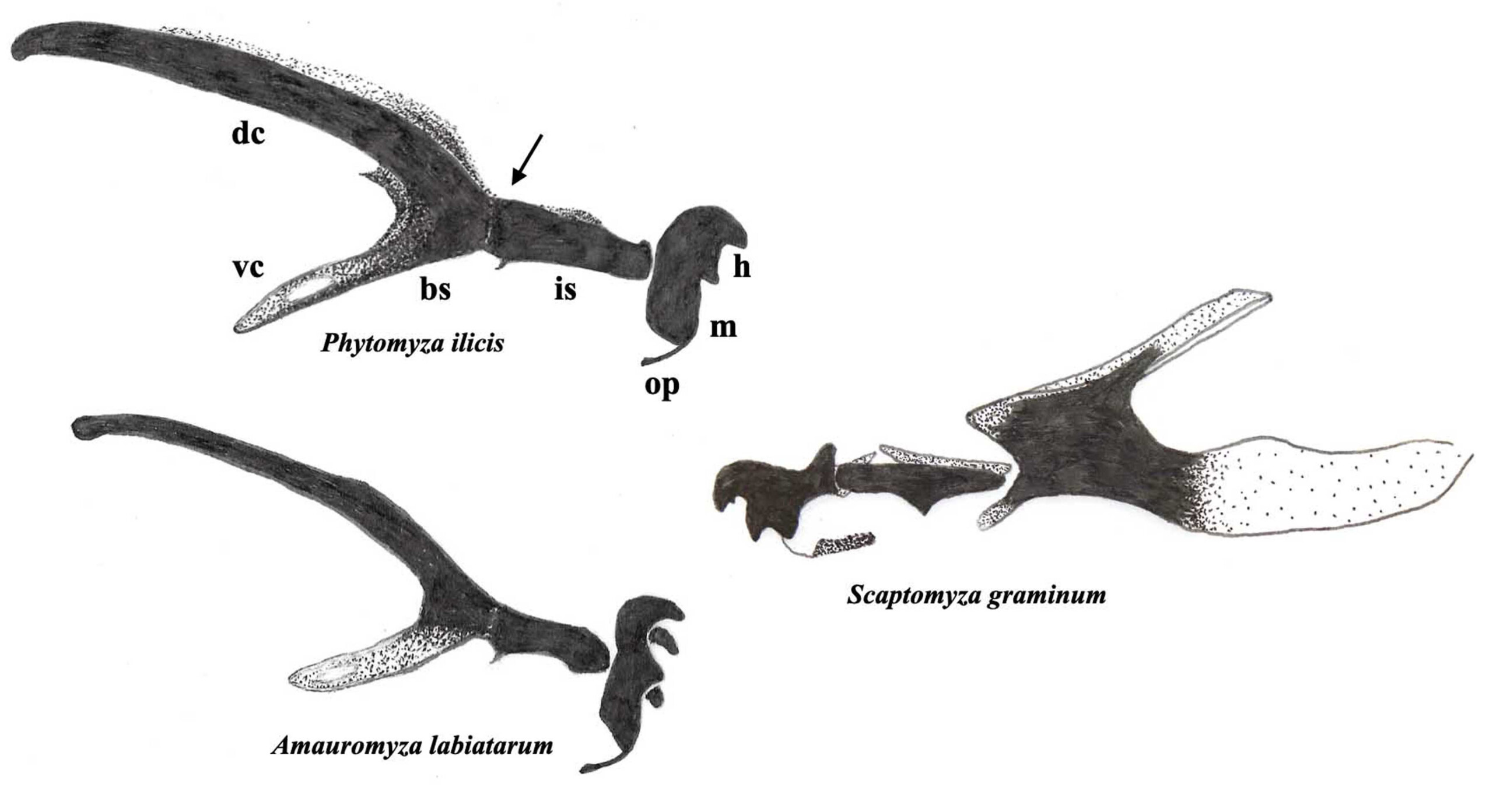
Fig. 1. Third stage larval head skeletons, profile views, Agromyzidae on the left and on the right, for comparison, leaf-mining Drosophilidae, arrow points to junction between the bs = basal sclerite and the is = intermediate sclerite; dc = dorsal cornu; h = mandible hooks; m = mandible; op = oral plate; vc = ventral cornu, more information in Rotheray (2019a).
Diagnosing morphology and finding distinguishing characters is one thing understanding how larvae live is another and that requires observations of living material. Realtime observations only go so far, videos are better. Videos provide a means of replaying behaviour at various speeds and forwards and backwards which makes it possible to work out the roles and relationships of morphological components. This in turn leads to an understanding of the mechanisms and patterns of feeding, locomotion etc (Rotheray 2019a). Leaf mining larvae can be videoed using digital cameras on their own or attached to binocular microscopes. Much of what follows was obtained using this method.
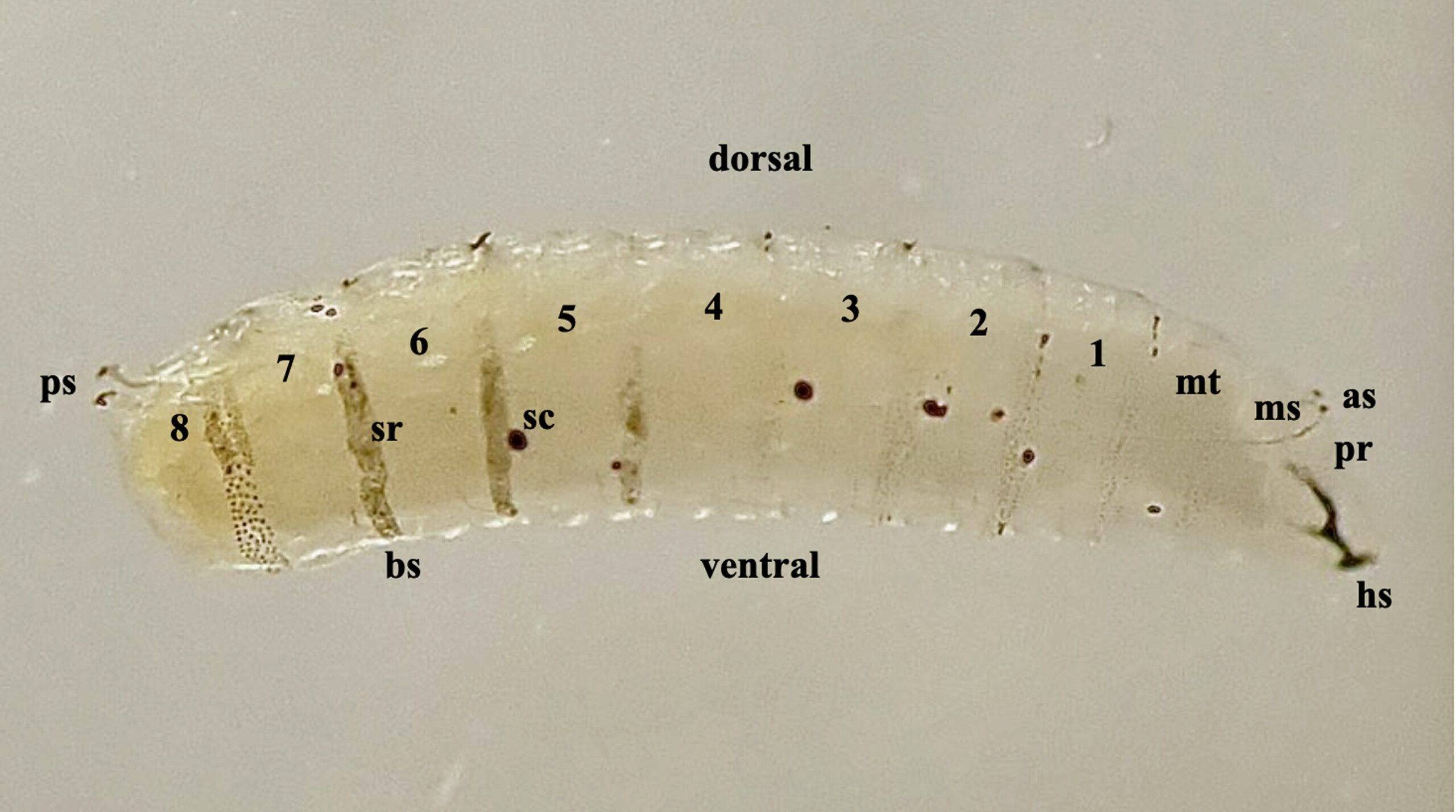
Fig. 2. Third stage larva of Amauromyza labiatarum, profile view, numbers 1-8 are abdominal segments; as = anterior spiracles; hs = head skeleton; ms = mesothorax; mt = metathorax; pr = prothorax; ps = posterior spiracles; sc = scar, the result of a wound to the body wall; sr = spicule ring.
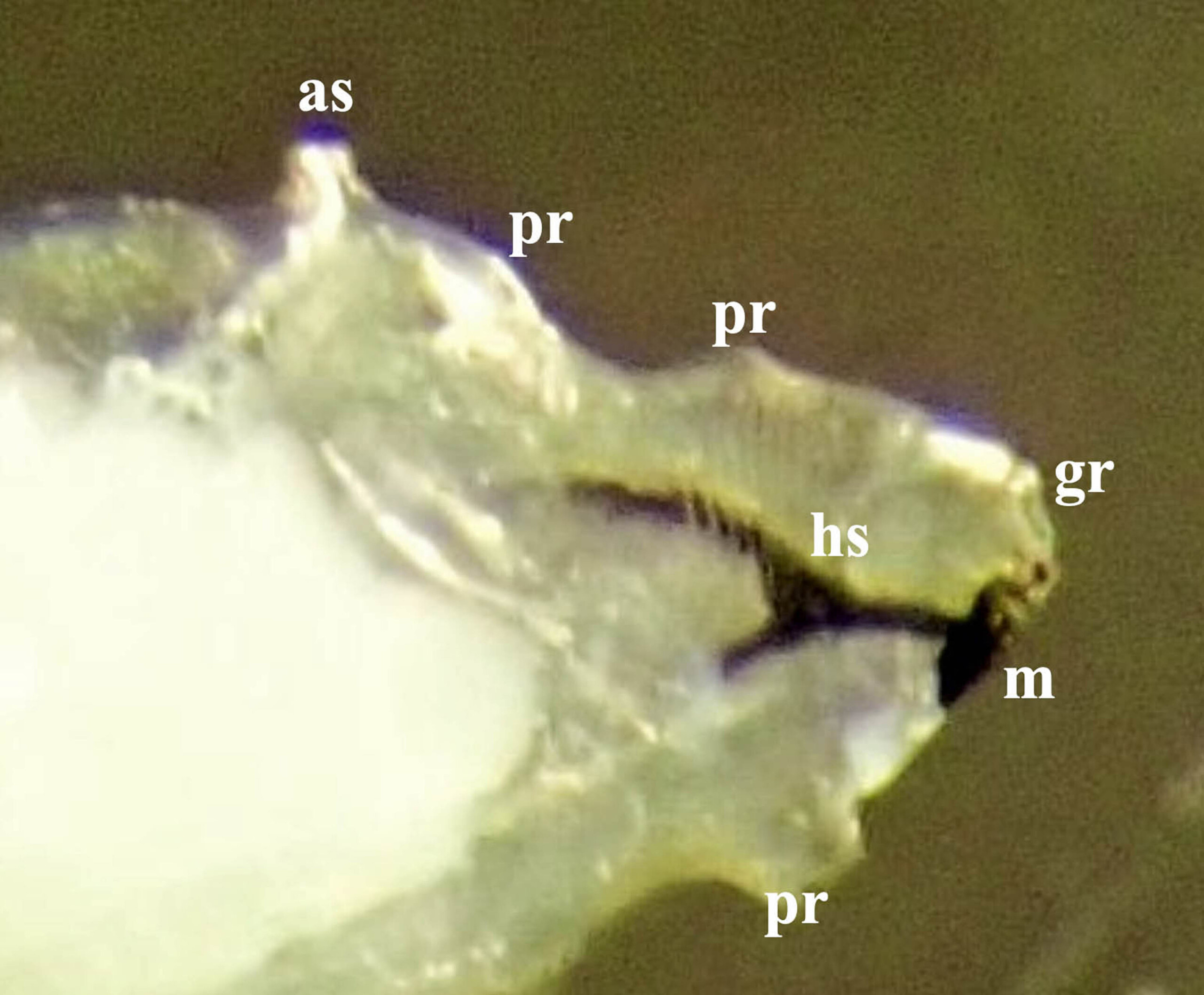
Fig. 3. Third stage larva of Amauromyza labiatarum, prothorax, profile view, pale mass to the left is body fat; as = anterior spiracles; gr = groove between the antennomaxillary organs; hs = head skeleton; m = mandible; pr = fleshy projections.
Feeding
To feed, most cyclorrhaphan larvae use their mandibles to gather food in front of the mouth from where a pump in the head skeleton sucks it through to the gut. The biomechanics of this process and the conditions under which food is accessed are major determinants of larval morphology (Rotheray 2019a). Essentially, feeding structures are optimised for demand, i.e. the physical forces required to gather food, that vary from low demand watery or soft food to high demand compacted or hard food. Locomotion, body shapes and vestiture or coverings are optimised for reaching food that varies from being in open to confined to closed spaces. Agromyzid larvae feed on food towards the hard and closed ends of these continua and as with other larvae feeding in these circumstances, they possess a fragmentation feeding mechanism.
Fragmentation involves a head skeleton that pivots up and down (Fig. 4). On the down stroke the mandibles move across a food surface and abrade it. These movements contrast with larvae gathering low demand food, such as saprophagous larvae feeding on watery decay, whose head skeletons move forwards and backwards. On the backwards stroke, the mandibles lower into food and drag a portion towards the mouth (Rotheray and Lyszkowski 2015).
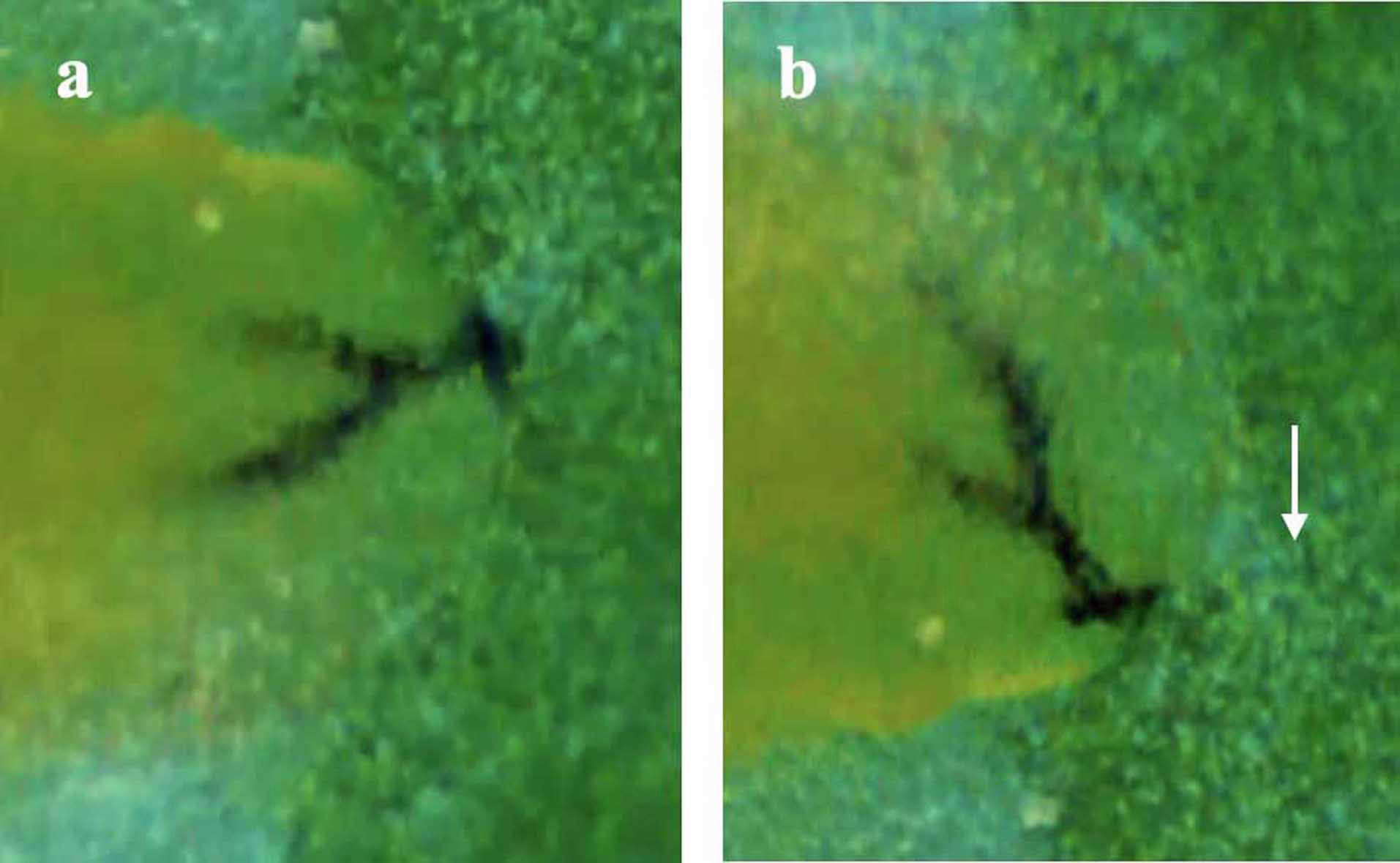
Fig. 4. A single head skeleton pivot in a third stage larva of probably, Phytoliriomyza melampyga, arrow indicates direction of pivoting, stills from a video recorded by Geoffrey Wilkinson in July 2016; a, start position; b, end position about a third of a second later.
In agromyzid larvae certain features associated with fragmentation are modified and additional ones are present:
- sclerotised groove present between the antennomaxillary organs at the front of the head (Fig. 3), fully illustrated in Ferrar (1987) and images in Dempewolf (2001);
- mandibles fused together (Dempewolf 2001, Rotheray 2019b);
- mandibles with little independent movement or fixed and unable to move (Rotheray 2019b);
- hooks of the two mandibles arranged alternately, one above the other, rather than the typical arrangement of being paired opposite each other (Dempewolf 2001, Rotheray 2019b);
- prothorax with a distinctive profile due to fleshy lobes and projections including one that supports the anterior spiracles (Fig. 3);
- cross-sectional shape of the abdomen elliptical, like an egg (Fig. 2).
The significance of these features is revealed when feeding and locomotion is compared with leaf mining larvae of other families, such as the Drosophilidae and Anthomyiidae (Rotheray 2019b). Forward-facing, alternately arranged mandible hooks are effective for reaching and fragmenting thin layers of leaf tissue and since the muscles that raise and lower the mandibles are reduced or absent, feeding is sustained with relatively low expenditures of energy. Most leaves are too narrow for leaf mining Diptera to complete feeding in an upright position and this probably explains why they lie on their sides to feed where the elliptical shape of the agromyzid abdomen optimises the amount of spicule-coated body surface gripping the upper and lower sides of the mine. With the rear end gripping, the front of the body is able to manoeuvre without the larva slipping and third stage agromyzid larvae are able to feed with it bent and contorted over an exceptional range of angles and shapes (Fig. 5). This means there is less need to alter position during feeding which helps maintain high feeding rates and minimal expenditures of energy.
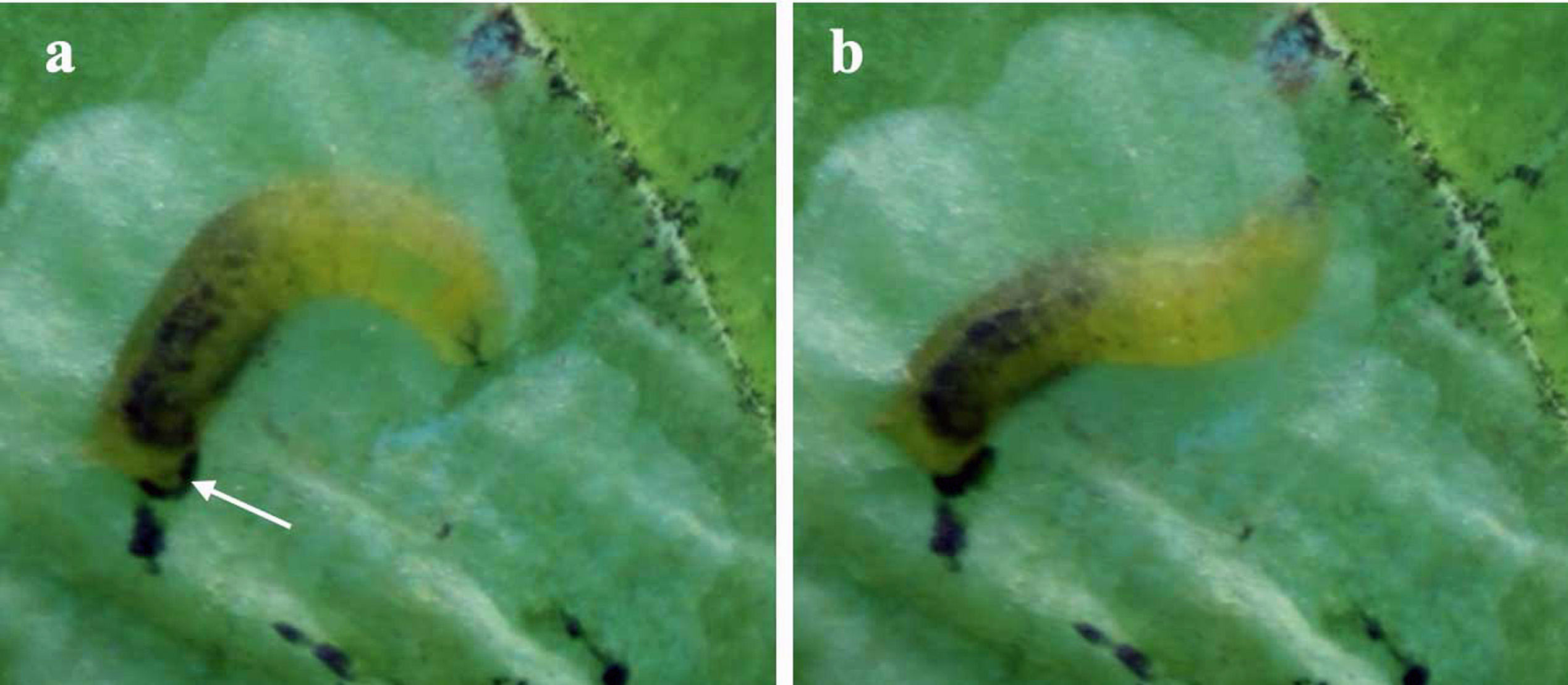
Fig. 5. Third stage larva of Phytoliriomyza melampyga, stills from a video recorded by Geoffrey Wilkinson, body positions during feeding, a, gripping with the rear end, lunging from the front, arrow points at an anal lobe surrounded by black frass; b, a few seconds later, the start of a new lunge.
To prevent the build up of pressure that would interfere with sucking, air or fluid passes along the groove between the antennomaxillary organs. The fleshy lobes on the prothorax probably help contain loosened food and prevent the anterior spiracles becoming inundated and blocked. Their raised, mid-dorsal position at the back of the prothorax further helps manage this risk. Taken together, these modifications facilitate a high level of feeding efficiency which appears to set agromyzid larvae apart from other leaf-mining Diptera. It defines them as specialists in their way of life and is, no doubt, a major factor facilitating plant colonisation.
Mine shape
A single pivot removes a thin line of tissue (Fig. 4). Pivots are usually made in a continuous series defined here as a lunge. With the rear of the body gripping the upper and lower sides of the mine the length of a lunge is governed by how far the front of the body bends and reaches food (Fig. 5a). At the end of a lunge the front of the body lifts to start a new one (Fig. 5b). Mines are extended by successive lunges and they often form species-specific shapes based on two general types, linear and blotch. Some mines shift between these two shapes, others are exclusively one or the other and some start out linear and change to a blotch. Linear mines are made by larvae removing tissue at the head of the mine by turning over at regular intervals and making alternate lunges on each side. Turning over is sometimes revealed by frass deposited alternately each side of the mine (Fig. 6). A blotch forms when larvae lunge without turning over. When sufficient space has been cleared they move into it, orientate perpendicularly to a mine face and clear successive, bowl-shaped scoops (Fig. 5b). In some cases, blotch mines result from linear mines coalescing, but it is unclear if this is more than coincidence.
In species that switch from linear to blotch mining, linear mines are the work of larvae up to about the early second stage and videos show that linear mines are maintained because their lunges are made at shallower angles than older, more developed individuals. This may reflect a lack of ability or strength in early stage larvae and raises the possibility that above a certain size or developmental stage, all leaf-mining larvae are capable of blotch mining. If so, the fact that many retain linear mining throughout development requires explanation. Also unclear is whether any first stage agromyzid larvae feed in an upright position and turn sideways when they reach a body size that is too large for upright lunging. Size-related shifts from upright to sideways feeding occur in, for example, leaf-mining Pegomya larvae (Anthomyiidae) (Rotheray 2019b).
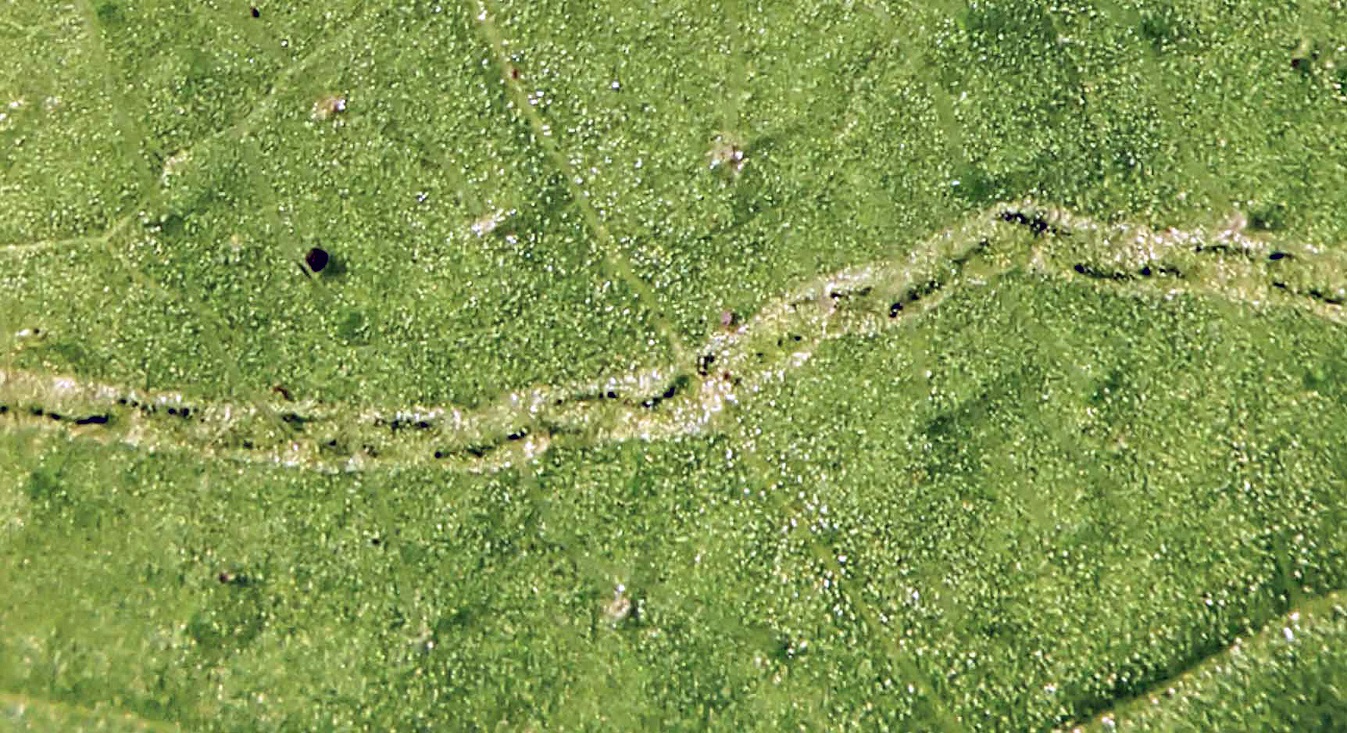
Fig. 6. Amauromyza sp., frass pattern in a section of linear mine, from Rotheray (2019b).
Systematic strip-mining sometimes occurs in blotch miners. Larvae initiate it by orientating perpendicularly to a mine face and making a scoop. This is gradually extended on one side by successive lunges and the larva gradually curves its body into the space so created, see Rotheray (2019a) for images of the process in the leaf-mining larva of Pegomya solennis (Anthomyiidae). A characteristic herring-bone pattern of remnants is often left behind in strip-mining (Fig. 7). Strip-mining also occurs in the Tephritidae and if all blotch mining larvae are capable of it, this is unclear (Rotheray and Lyszkowski 2015). Casual observation suggests that feeding rates are higher in strip than non-strip mining. High feeding rates appear to be due to more continuous feeding, i.e. fewer and shorter interruptions to adjust position, but further investigation is needed.
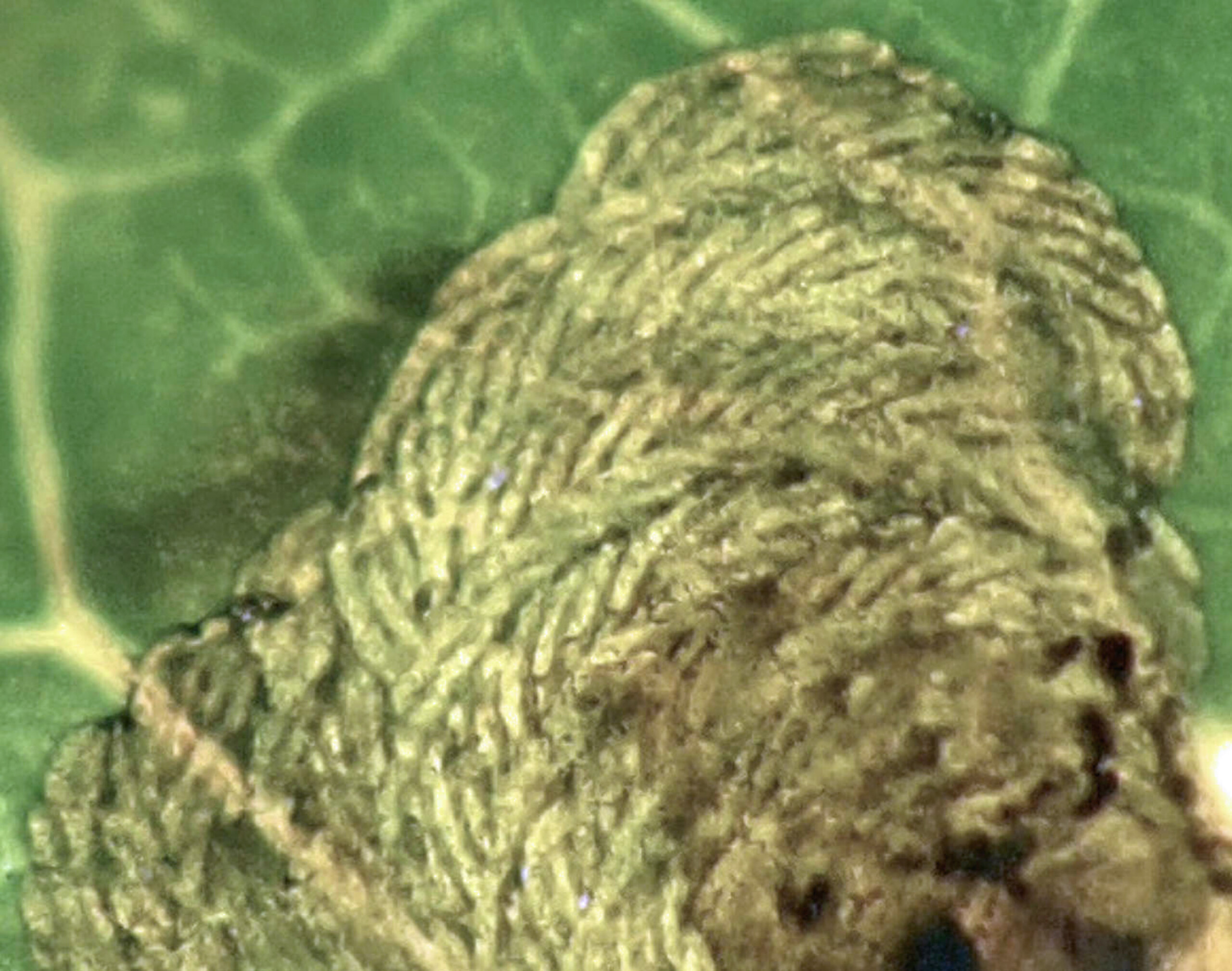
Fig. 7. Phytomyza ilicis herring-bone pattern of feeding remnants, from Rotheray (2020).
Although leaf mining agromyzid larvae use a fragmentation feeding mechanism which itself is a modification for feeding on high demand food, leaf tissues vary in density and agromyzids appear to adapt accordingly. For instance, holly leaf tissue (Ilex aquifolium, Aquifoliaceae) is dense, thick and tough relative to leaf tissue in red campion (Silene dioica, Caryophyllaceae) and woundwort (Stachys sp., Lamiaceae) (Rotheray 2020). Certain contrasts occur between the holly leaf miner, Phytomyza ilicis, and the leaf miners, Amauromyza flavifrons and Amauromyza labiatarum that mine red campion and woundwort respectively. For instance, the larva of P. ilicis has larger mandibles with shorter, wider hooks that are paired opposite one another not arranged one above the other, a larger more sclerotised head skeleton and sclerotisation in the mandible-supporting, oral cavity at the front of the head (Fig. 1) (Rotheray 2020). By analogy with larvae specialised for low and high demand food in the families, Drosophilidae and Lonchaeidae, these contrasts are consistent with optimisation for different levels of tissue density (Rotheray 2019a).
Such fine-level differences could easily be dismissed as trivial variation, but observations and feeding assessments show otherwise and are a reminder that without assessment, nothing should be assumed. Greater sclerotisation in the P. ilicis head skeleton and oral cavity and shorter, wider mandible hooks appear to reflect greater requirements for buttressing due to the denser nature of holly leaf tissue and the pairing of mandible hooks may be a response to its greater thickness. Herring-bone feeding remnants in P. ilicis mines are also relatively compressed and mines are smaller than those of the Amauromyza species (Rotheray 2020). Compressed remnants might be a consequence of tissue that takes more effort to fragment and a higher tissue volume per unit area may explain the smaller mines. Such contrasts are unlikely to be confined to these three species, but to some level, characteristic of their respective lineages. The influence of other factors possibly effecting mine size have not, however, been eliminated, for example, whether smaller P. ilicis mines reflect a smaller larva or one with lower nutritional requirements. Investigation of the effects of tissue density on larval feeding are far from exhausted and examples elsewhere will provide additional insight, such as the remarkable and apparently extreme modifications found in the body shape and mandibles of cambium-mining Phytobia larva (Agromyzidae) (Ferrar 1987). Another set of potentially revealing contrasts relate to food tissues arranged in other ways than occur in leaves, such as in seeds, stems, galls etc.
The relative hardness of leaf veins can also have an effect, at least on mine shape. Although some agromyzid larvae mine through leaf veins, others are influenced by contact with large veins and change direction or mine alongside them. This seems to depend on the angle of contact with oblique approaches tending to deflect mining and perpendicular ones having no effect. This is probably due to veins obstructing feeding lunges more in oblique than perpendicular contacts, but this needs further study. Agromyzid larvae are far from the point of being ‘mined out’ of taxonomic and ecological information. As we’ve seen, a major biomechanical interaction occurs between agromyzid larvae and their foodplants. It exists in the variable ways agromyzid larvae do the physical work of mining and evidence for it has only just begun to be acquired. Agromyzid larvae certainly deserve to be viewed as more worthwhile of time and attention than as mere passing stages in rearing processes whose ultimate objective is solely that of obtaining adults.
References
Dempewolf, M. 2001. Larvalmorphologie und Phylogenie der Agromyzidae (Diptera). Unpublished Ph.D thesis, Universität Bielefeld, Germany.
Ferrar, P. 1987. A guide to the breeding habits and immature stages of Diptera Cyclorrhapha. Entomonograph 8,1-907.
Hering, E.M. 1951. The Biology of the Leaf Miners. Junk, Gravenhage.
Rotheray, G.E. and Lyszkowski, R.L. 2015. Diverse mechanisms of feeding and movement in Cyclorrhaphan larvae (Diptera). Journal of Natural History 49, 2139-2211.
Rotheray, G.E. 2019a. Ecomorphology of Cyclorrhaphan Larvae (Diptera). Zoological Monographs 4. Springer. Heidelberg, Germany.
Rotheray, G.E. 2019b. Mechanisms and patterns of feeding in some leaf-mining larvae (Diptera, Agromyzidae, Drosophilidae and Anthomyiidae). Dipterists Digest 26, 113-137.
Rotheray, G.E. 2020. Effects of tissue hardness on larval feeding in the holly leaf miner, Phytomyza ilicis Curtis (Diptera, Agromyzidae). Dipterists Digest 27, 143–154.
Smith, K.V.G. 1989. An Introduction to the Immature Stages of British Flies. Handbooks for the Identification of Britsh Insects. 10, 1–280.